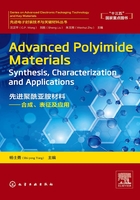
2.3 Preparation of Polyimide Fibers
At present, three methods are generally employed to fabricate PI fibers including melt-spinning, electro-spinning, and solution spinning. The solution spinning includes dry-spinning, wet-spinning, dry-jet wet-spinning, and liquid crystalline spinning. In the following parts, introduction of the above-mentioned methods is expounded, combined with specific processing characteristics and applications.
2.3.1 Wet-Spinning Method
Wet-spinning is one of the most widely used methods for the fabrication of PI fibers. A diagram of the wet-spinning process is shown in Fig. 2.6; the spinning solution is extruded into the coagulation bath through the spinneret, and then the solvent in the spinning solution and the non-solvent in the coagulation bath interdiffuse, which results in the phase separation. In this situation, polymer dense phase and dilute phase appear in solution, and filament turns into solid fiber gradually along with phase separation. The wet-spinning process can be divided into “one-step” and “two-step” methods according to the differences in the prepared spinning solutions. In the “two-step” wet-spinning process, PAA solution is spun into precursor fibers firstly, and then PI fibers can be obtained through the chemical or thermal imidization. In the early research, the “two-step” method was generally thought to be disadvantageous for preparing PI fibers with high strength and modulus due to the volatilization of water that existed in as-spun fibers always leads to microporous defects in PI fibers during the complicated thermal imidization process, which weakens mechanical properties of the final fibers. However, with the development of synthesis and spinning technologies, the above statement is considered to be immature. In the “one-step” wet-spinning method, as-spun PI fibers can be directly obtained from the spinning solution of soluble PI. Therefore, microporous defects are avoided without volatilization of water existing in as-spun fibers, and it is in favor of aggregation structure controlling, which is beneficial for the fabrication of PI fibers with high strength and modulus.

FIGURE 2.6 Diagram of wet-spinning process.
2.3.1.1 “Two-Step” Wet-Spinning Method
In the “two-step” wet-spinning process, defects generated in the fiber-forming process and complicated post-process due to volatilization of water existing in as-spun fibers are the main pivotal problems, which obstruct the thermal stretching process and finally weaken the final performance of fibers, so fabrication of as-spun fibers with a dense structure is very important. For this purpose, solving the problem of the formation of defects in as-spun fibers becomes a key point in improving the performance of PI fibers and an extensive discussion on controlling the micromorphology of as-spun fibers has been held at home and abroad. Goel et al. [29,30] synthesized a type of PAA from 4,4′-diaminodiphenylmethane (MDA) and PMDA in DMF and then PAA fibers were produced from the mixed coagulation bath of DMF and H2O. After chemical imidization treatment (Vaceticanhydride: Vpyridine=1:1), thermal stretching process was carried out at 300 °C. The tensile strength of the obtained PI fibers only reached about 0.05 GPa because of the existence of many micropores in fibers and the thermal stretching process made the pores even larger according to the SEM images, which proves the importance of the morphology adjustment in the fabrication process of as-spun fibers. Dorogy and Clair [31] discussed the effects of coagulation bath conditions on the fiber-forming process based on the BTDA/ODA/DABP (3,3′-diamino- benzophenone) system in detail. They analyzed the pores in as-spun fibers produced in the mixed coagulation bath of H2O and ethanol, H2O and ethylene glycol, H2O and DMAc. The cross-section of the resulting fibers was the most dense when the coagulation bath was a mixture of 80% ethanol/H2O solution or 75% ethylene glycol/H2O solution, but many microporous defects were generated when using the other coagulant.
Park and Farris [32] prepared a type of PI fiber with a dense structure by the wet-spinning method from the partial imidization PAA solution and thermal imidization process. The tensile strength and modulus of final fibers were 0.4 GPa and 5.2 GPa, respectively. However, compared with the pure PAA spinning solution with no imidization, the partially imidized PAA spinning solution has lower diffusion velocity, which is helpful for forming denser structures in the resulting fibers and avoiding the formation of skin-core structure. Meanwhile, this study provides a new method for the high draft in the dry-jet wet-spinning process, because PAA fibers cannot bear the pressure from the high draft. Zhang and coworkers [33] analyzed the dual diffusion process of the PAA solution in H2O, ethanol, and ethylene glycol in detail, based on available theoretical and experimental ternary phase diagrams to optimize the fabrication process of PI fibers by the “two- step” wet-spinning method. As shown in Fig. 2.7, solidification abilities of the three kinds of solvents were H2O>ethylene glycol>ethanol. It is noteworthy that the interaction parameter of the ethanol/PAA system was only 0.28 which is extremely small. This result illustrated that the solidification ability of ethanol was relatively weak compared with the other two solvents, and H2O showed the strongest solidification ability. In the three non-solvents, the polymer contents at the dividing point were all under 5%(w), thus the forming process of PAA tended to be by the nucleation growth, which is helpful to produce as-spun fibers with dense structures.

FIGURE 2.7 Interaction parameter of PAA/DMAc and coagulant/PAA system at 27 °C.
In recent years, with the development of synthesis and spinning technologies, the integrated performance of PI fibers fabricated by the “two-step” wet-spinning method was improved constantly. Inspired by the heterocyclic Aramid fiber named Armos from the Soviet Union Tver Chemical Fiber Freedom Company, introducing heterocyclic moieties into PI main chains via heterocyclic diamine monomers and fabrication of high-performance PI fibers by the “two-step” wet-spinning method has become a new research hotspot, and achievements are prominent. Liu et al. [34] carried on copolymerization from 2-(4-aminophenyl)-5-aminobenzimidazole (PABZ)/ PMDA/ ODA and fabricated high-performance PI fibers by the wet-spinning method. As shown in Table 2.1, the monomer ratio of PABZ and ODA had a critical influence on the mechanical properties of PI fibers. When the monomer ratio was PABZ/ODA=7/3, tensile strength and modulus of PI fibers were enhanced about 2.5 times and 26 times, reaching 1.53 GPa and 220 GPa, respectively. According to the test results of FTIR and DMA, strong intermolecular hydrogen bonding existed in the fibers owing to the introduction of PABZ, which was much helpful for the improvement of fibers’ mechanical properties.
TABLE2.1 Mechanical Properties of PMDA/ODA/PABZ PI Fibers

A series of heterocyclic PI fibers were prepared from BPDA/PPD/BIA (BIA: 2-(4-aminophenyl)- 5-aminobenzimidazole) and BPDA/PPD/PRM (PRM: 2,5-bis(4-aminophenyl)-pyrimidine), and many deep studies on some critical point were carried out, such as fiber’s structure, mechanical properties, thermal stabilities, and so on.
In recent years [35-40], a series of PI and co-PI fibers have been produced through the integrated continuous two-step wet-spinning method, and the chemical structures are shown in Fig. 2.8. In their studies, the BPDA/PDA homo-PI fibers possessed tensile strength, initial modulus, and elongation of about 1.0 GPa, 50 GPa, and 1.1%, respectively, attributing to the rigid chain structure. However, the stiffness of the polymer chains also results in poor spinnability and low mechanical properties of the resulting fibers. Therefore, with the aim of improving the mechanical properties and processibility of the PI fibers, the most efficient approach is modifying the chemical structures of PIs by incorporating other moieties into the BPDA/PDA backbones. By optimizing the chemical structure and controlling the spinning conditions, the co-PI fibers exhibited tensile strength higher than 3.0 GPa and modulus higher than 130 GPa.

FIGURE 2.8 Typical chemical structures of copolyimide fibers prepared in Wu’s laboratory.
A new heterocyclic diamine monomer with high reactivity and rigid-rod structure named 2- (4-aminophenyl)-6-amino-4(3H)-quinazolinone (AAQ) has been synthesized, and was introduced into the main chain of PI to fabricate fibers with high tensile strength and modulus (Table 2.2). The results indicated that there were strong intermolecular hydrogen bonds in fibers since the introduction of AAQ monomer. In addition, orientation degrees of molecular chain and crystalline structures in fibers were much improved on account of the rigid-rod structure of the AAQ monomer, which is of much significance for improving fibers’ mechanical properties. They also introduced 4,4-oxydiphthalic anhydride (ODPA) and ODA containing ether groups into the BPDA/PDA polymer backbone, and discovered that the introduced ether groups were helpful for the reduction in the microvoids’ size in the fibers. By combining the advantages of BIA and ODA units, a series of BPDA/PDA/BIA/ODA co-PI fibers have been prepared. The mechanical properties of the co-PI fibers were improved due to the reduction in the microvoids and strengthened intermolecular interactions, respectively.
TABLE2.2 Mechanical Properties of Polyimide Fibers From BPDA/AAQ/PDA [39]

In conclusion, PI fibers with high strength and modulus can be fabricated by the introduction of heterocyclic units into PI macromolecular chains through the “two-step” wet-spinning method. Some common heterocyclic diamine monomers and their fibers’ performance are listed in Table 2.3.
TABLE2.3 Common Heterocyclic Diamine Monomers and Their Fibers’ Performance

2.3.1.2 “One-Step” Wet-Spinning Method
The appearance of soluble PIs promotes the development of fabrication technologies based on the “one-step” spinning method for PI fibers. In traditional “one-step” wet-spinning or dry-jet wet-spinning processes, phenolic solvents (m-cresol, p-chlorophenol, m-chlorophenol) were widely used, and alcohol (methanol, ethanol, and ethylene glycol) or mixture of alcohol/water were generally used as coagulants. PI fibers with high tensile strength and modulus can be obtained by high draft of as-spun PI fibers. In an early research, Kaneda et al. [43] from Kyoto University synthesized a series of soluble PIs in p-chlorophenol solvent based on BPDA and various diamine monomers, and the PI fiber with a tensile strength of 3.1 GPa and modulus of 128 GPa were prepared by “one-step” wet-spinning in the coagulation bath of ethanol/H2O mixture. Moreover, compared with Kevlar 49 from DuPont, the above fibers have lower water absorption and stronger acid resistance. Cheng’s group [44] from Akron University also prepared PI fibers in methanol/H2O coagulation bath from soluble PI/m-cresol solution with a content of 12%-15%(w) synthesized from BPDA and 2,2′-bistrifluoromethyl-4,4′- diaminobiphenyl. After a high draft of 10 times at 380 °C, the tensile strength and modulus reached 3.2 GPa and 130 GPa, respectively. Furthermore, the modulus of obtained fibers reduced only 5% after treatment at 400 °C for 5 h, which illustrated the outstanding high-temperature strength retention of the prepared PI fibers. As mentioned earlier, the “one-step” method is beneficial to prepare PI fibers with high tensile strength and modulus. However, phenolic solvents are usually hypertoxic and difficult to thoroughly remove from the as-spun fibers. Therefore, if PIs with special structures and high organo-solubilities are synthesized, the “one-step” fabrication process of PI fibers is more promising. As reported by Zhang and coworkers [27], a series of co-PIs were synthesized from BTDA, 2,2′-bis(trifluoromethyl)-4, 4′-diaminobiphenyl (TFMB) and BIA with different diamine ratios via the “one-step” polymerization at 190 °C in the NMP solvent, and the inherent viscosity and number-average molecular weight of the obtained PI/NMP solution were 1.83-2.32 dL·g−1 and 31,300-41,000, respectively. And then, a series of high-performance PI fibers whose tensile strength, modulus, and elongation at break were 1.37 GPa-2.13 GPa, 29.9 GPa-101.9 GPa, and 4.57%-2.14%, respectively, were fabricated by a “one-step” wet-spinning and thermal stretching process. These works provide important references for preparing high-performance PI fibers by the “one-step” wet-spinning method in common and environmentally friendly organic solvents. Chen et al. [4] also have successfully synthesized a type of heterocyclic PI with an inherent viscosity of 6.98 dL·g−1 by “one-step” method at high temperature in PPA, and the synthetic route is shown in Figs. 2.9. In the meantime, a series of PI fibers with high strength and modulus were prepared by dry-jet wet-spinning and their tensile strength were in the range of 2.45 GPa-3.12 GPa. Compared with phenolic solvents, PPA is more environmental friendly with low toxicity, and PI can form liquid-crystal state in PPA, which is in favor of obtaining high-performance fibers.

FIGURE 2.9 Synthesis route of poly(imide-co-benzoxazole) by “one-step.” Polymerization at high temperature in PPA.
2.3.2 Dry-Spinning Method
Fibers prepared by the dry-spinning process are based on a specific fiber-forming principle, which is different from the melt-spinning or wet-spinning method. In the melt-spinning process, polymer melts are gradually solidified along the spinning line with the decreased temperature. PI fibers are difficult to fabricate by the melt-spinning method due to the rigid backbones and strong intermolecular interactions of PI chains, which result in the unmelt behavior of PIs even under a high temperature. In the wet-spinning, the coagulation of as-spun fibers is a dual diffusion process, namely, the solvent in the solution diffuses into the coagulation bath and the non-solvent diffuses into the fibers, which is a relatively complex process. For the dry-spinning method, fibers are quickly solidified due to the rapid evaporation of solvent in the spinning solution and the spinning process is easily affected by the temperature and volume of air in the column. So far, several commercial fibers, including polyurethane and cellulose acetate fibers, have been successfully prepared by the dry-spinning process. Compared with the wet-spinning, advantages of the dry-spinning process are as follows: (1) higher spinning rates, more environmentally friendly, and more convenient for the solvent recovery; (2) the spinning solution in the hot column of the dry-spinning process will solidify rapidly by an unidirectional diffusion of the solvent, which is beneficial for the formation of denser inner structures of the resulting fibers, as shown in Fig. 2.10. However, the prerequisite of polymeric solutions for the dry-spinning is much harsher, for example, the apparent viscosity of the solution should be fit for the spinning conditions, the rheological properties should be good enough, and its higher requirements on equipment compared with the wet-spinning process.

FIGURE 2.10 (A) SEM pictures of the cross-section for the as-spun PAA fiber by dry-spinning, (B) thermally imidized PI fiber, (C) drawn PI fiber with Dr=2.5, and (D) cross-section of the as-spun PAA fiber with the same backbone by the wet-spinning method.
Early studies about PI fibers prepared via the dry-spinning process started in the 1960s. Investigators in DuPont were aware of the outstanding combination of properties of PI fibers, and then they tried to produce high-strength and high-modulus PI fibers via dry-spinning, and they applied for corresponding patents. Samuel and Edgar [45] successfully synthesized a PAA spinning solution with the inherent viscosity of 1.3 dL·g−1, and they attempted to prepare PI fibers using this solution in a spinning column at 265 °C with a spinning rate of 160 m·min−1. The final tensile strength and modulus of the PI fiber were around 3.5 g·d-1and 50 g·d−1, respectively. About 20 years later, researchers in Dupont tried to prepare co-PI fibers via the dry-spinning process again by using 3,4′-ODA and p-PDA as the diamines, and PMDA as the dianhydride. The tensile strength and modulus of the final co-PI fibers reached 15.6 g·d-1 and 534 g·d−1 after treating the precursor co-PAA fibers under 150, 200, and 300 °C for 20, 20, and 30 min, respectively [46]. Fig. 2.11 depicts the schematic diagram of preparing PI fibers by dry-spinning process. Here, a brief description is given in the following to introduce some characteristics of this spinning method.

FIGURE 2.11 Schematic diagram of preparing PI fibers via dry-spinning.
In general, the solvent in the spinning solution will evaporate rapidly, which is attributed to the great difference of temperature between the hot air in the column and the spinning solution. That is, the solvent will undergo a flash process resulting in the solidification of polymer solutions. In the beginning, the spinning solution contains large quantities of solvents (around 80%-90%(w)), and the diffusion rate of the solvent in the filament is mainly controlled by the heat and mass transfer rate. Solidified filament forms in the one-third part of the spinning line, and the evaporation rate of solvent decreases to around zero. In fact, the strong hydrogen bonding interaction can form between PAA and the solvent (like DMF, DMAc), and the residual solvent is difficult to fully remove due to the fact that the filament stays for a short time in the spinning column. As shown in Fig. 2.12, the residual solvent content in the as-spun fiber is closely related to the spinning speed, which dramatically increases with increasing spinning speed, and the final content is mainly in the range of 15%-30%(w), this residual solvent plays an important role in the following thermal imidization and hot-drawing process of the fibers [47].

FIGURE 2.12 The content of the residual solvent in as-spun fibers as a function of the spinning speed.
There is a big difference in the dry-spinning process between PI fibers and other polymeric fibers: the precursor PAA will be partially thermally imidized to PI in the high-temperature spinning column. As Fig. 2.13 shows, the imidization process for the PAA in the spinning column can be divided into two different stages along the spinning line: in the first stage, the imidization degree changes little due to the relatively low temperature of the filament because the rapid evaporation of the solvent will take away a great deal of heat; in the late stage, the imidization degree increases quickly due to the raised temperature of the solidified filament. The partial imidization of the PAA fiber shows a great significance for its storage stability and the following thermal treatment. Meanwhile, the imidization degree shows a higher value under low spinning speeds due to the relatively long imidization time.

FIGURE 2.13 The imidization degree of as-spun fibers as a function of spinning line length and spinning speed.
Zhang and coworkers have made a great efforts on the preparation of PI fibers by dry-spinning method [48-50]. In order to investigate the partial imidization reaction of PAA in the spinning column, a dry-spinning model for the PI fibers was established. Interestingly, by building corresponding spinning equations, a credible model for spinning profiled PI fibers was successfully obtained. Herein, a brief introduction was given.
A trilobal cross-section of the spinneret hole and surface tension on the fiber with trilobal cross-section model were given as designed in Fig. 2.14, and the streamline diagram and velocity vector of the spinning solution in the designed spinneret were successfully simulated by the software of Polyflow. Near the spinneret, the filament contains a high amount of free solvent, and flash evaporation takes place at the high temperature of the air flow in the spinning column. The mass fraction of the DMAc thus decreases rapidly and the filament solidifies at z=150 cm, as shown in Fig. 2.15. At the same time and as a result of flash evaporation near the spinneret, the temperature of the filament decreases rapidly to a minimum at z=85 cm. It then quickly reaches the temperature of the hot air (253 °C) at z=290 cm.

FIGURE 2.14 (A) The designed spinneret with a trilobal cross-section; (B) the streamline diagram; and (C) velocity vector of the spinning solution in the designed spinneret.

FIGURE 2.15 Variation of solvent mass fraction and temp-erature along the spin line.
The formation of profiled PI fibers in the high-temperature column can be controlled by the spinning parameters (temperature, drawing ratio, hot air velocity). In the dry-spinning process model, the air velocity is changed from 20 cm·s-1 to 100 cm·s−1, as shown in Fig. 2.16. At the spin line z<120 cm, the profile degree drops due to the solvent evaporation and the viscoelastic behavior of the filament; the air velocity has no influence on the profile degree. At z>120 cm, a high air velocity results in a relatively high value of the profile degree. It must be attributed to the increased air velocity that results in higher rate of solvent evaporation. The filament shape is thus “freezed” at short times.

FIGURE 2.16 Effect of air velocity on profile degree.
The impact of air temperature in the spinning column on the profile degree is shown in Fig. 2.17. The final profile degree decreases for lower temperature; the maximum profile degree is declined at T=253 °C. As we know, the higher temperature leads to solvent evaporating more rapidly and the short solidification time of the filament. Besides, the high air temperature increases imidization reaction degree of PAA, causing a larger viscosity of spinning dope before solidification. Thus, the cross-section deformation under surface tension at high temperature reduces, and the final profile degree is larger compared to one at lower temperatures. Based on the above results, bringing the solidification point close to the spinneret helps to obtain (within certain limits) a larger final profile degree.

FIGURE 2.17 Effect of temperature in spinning column on profile degree.
By keeping the mass flow constant but increasing the take-up velocity, a larger draw ratio (DR) can be achieved. Fig. 2.18 shows the dependence of the profile degree on the DR from 1 to 3. A clear trend emerges: the profile degree increases with the DR. On the one hand, a high DR results in thinner filaments with a higher specific surface area, which accelerates solvent evaporation and solidification. On the other hand, a high DR increases the velocity of the filament and decreases residence time in the column, which goes against solvent evaporation. The combination of these two opposing effects results in a lager profile degree with the DR increase. The final value reaches in our simulation 0.24 at DR=1 and 0.40 at DR=3.

FIGURE 2.18 Effect of draw ratio on profile degree.
In summary, in the dry-spinning process, precursor PAA fibers are firstly solidified as the evaporation of the solvent and then partial imidization reaction takes place, which greatly enhances the stability of the PAA fibers. Therefore, the phase separation process and imidization reaction of the PAAs in the high-temperature column have a great effect on the microstructure, morphologies, and properties of the final PI fibers.
2.3.3 Other-Spinning Methods
2.3.3.1 Liquid-Crystal Spinning Process
In the liquid-crystal spinning process, the spinning solution will be subject to the strong shearing and stretching effects when extruded from the spinneret. The nematic liquid-crystal microregions will orient along the fibers’ axis, and the molecular chains will further orient in the air gap and will be fixed when the solution comes into the coagulation bath. Therefore, fibers spun via the liquid-crystal spinning process always show high strength and high modulus even without thermally stretching. Few studies about PI fibers fabricated by the liquid-crystal spinning have been reported due to the fact that soluble PAAs are difficult to form liquid-crystal phase. Neuber et al. [51] successfully synthesized a poly(amic ethyl ester) (PAE) solution with an inherent viscosity of 5.89 g·cm−3. This PAE/NMP solution can form lyotropic liquid-crystal phase when the solid content reached 40%(w) at 80 °C. Corresponding PI fibers were successfully prepared via the liquid-crystal spinning process using acetone as the coagulant. The final tensile strength and modulus of the fibers were 0.7 GPa and 68.0 GPa, respectively.
2.3.3.2 Melt-Spinning Process
Melt-spinning is a common spinning method to fabricate polymer fibers. However, PI fibers were rarely prepared by this spinning method due to low solubility and infusibility properties of most PIs because of their rigid backbones. However, more and more poly(ether imide)s have been developed in recent years by introducing flexible ether or methylene units into polymer chains that endow these PIs with good spinnability by the melt-spinning process. Researchers in Teijin Chemicals Ltd. tried to produce poly(ether imide) fibers via the melt-spinning process at temperatures of 345 °C-475 °C. However, the mechanical properties of the final fibers were unsatisfactory. Irwin [52] also tried to fabricate PI fibers via the melt-spinning process under 300 °C- 400 °C, and rolling speed reached 300 m·min-1-500 m·min−1. The tensile strength of as-spun fibers was just 0.59 GPa, and the final strength and modulus of fibers after thermal treatment reached 1.55 GPa and 48 GPa, respectively. Based on the above analysis, it’s easy to conclude that the melt-spinning temperature of PI fibers is always too high, yet the prerequisite of this spinning method is that PIs can be melted at certain temperatures. These limitations mean that the melt-spinning process is not suitable for preparing PI fibers on a large-scale.